In our last post What is a Wide Area Blackout?, we defined a wide area blackout as an incident in which part of the electric power transmission system collapses, interrupting the flow of electric power to 250,000 customers or more. Though rare, wide area blackouts have costly impacts.
In this post, we will explore the three key triggers of a wide area blackout: load level, fault type, and fault clearing time. This post gets a bit technical, so feel free to contact us if you have any questions.
The Uphill Climb
The electric power grid can be compared to a fully loaded, ten wheel dump truck going uphill at 60 mph. The instant that the driver lifts his foot off the gas pedal, the truck slows down. Reacceleration is problematic. The conceptual difference is that the electric power grid is always climbing “uphill.” Sometimes the slope is less than 1%, sometimes the slope is more than 25%, but the slope is always in an upwards direction. Even when the load is ramping down, the power system is still continuing an uphill climb.
Routine changes in customer load are ramp-like increases, as illustrated in Figure 1. The figure shows typical daily load profiles for the electric power grid over a 24 hour period. During a typical summer day, between the hours of 5:00 am to 11:00 am, the amount of power being demanded is increasing continuously, as a ramp increases in height, rather than in large chunks, like steps.
This is typical of the electric power grid; the grid is designed to be resilient as power demand is increased in a ramp-like manner. However, if the power grid is to rebound when a triggering event occurs, it must be able to overcome step-like changes in load.
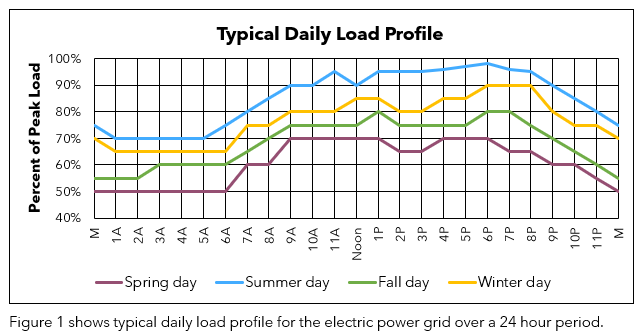
Power Transfer Concepts
Figure 2 shows a visualization of power transfer. One hundred percent of energy produced by rotating generators is consumed by motors and other devices, and the electric power grid is balanced. As energy demand “ramps up” each day, energy production is increased to match the demand. (One keynote is that the rotational speed of motors lags behind the rotational speed of energy producers by a few percent).
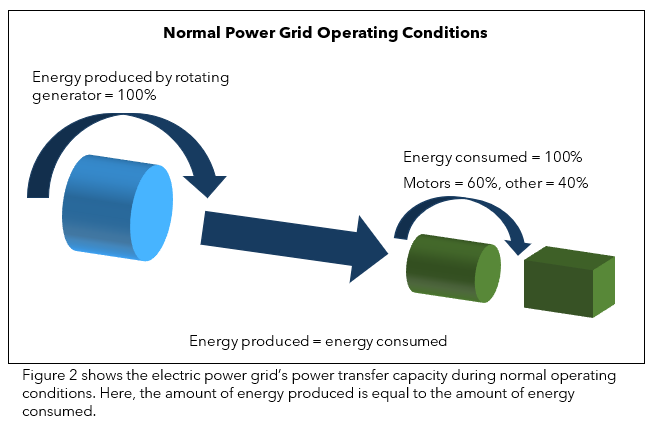
When faults or short circuits occur, the link between energy sources and energy consumers is distorted, and the amount of energy delivered to consumers is reduced. This is illustrated in Figure 3. The result is that rotating energy sources (traditional generators) start accelerating, while motors start decelerating.

Wide area blackout analysis should focus on three phase fault conditions, because when a three phase fault occurs, the entire power grid is disturbed. In contrast, when a single phase fault occurs, one third of the power grid is disturbed.
During fault conditions, generators accelerate because input power is unchanged while output power is reduced. The difference between input energy and output energy becomes accelerating energy. Motors start decelerating because input power is reduced while shaft power remains unchanged.
When a Fault Occurs: Important Considerations
Fault duration is an important consideration at this point. The power grid operates at 3600 RPM while motors operate at various speeds, including: 3600 RPM, 1800 RPM, 1200 RPM or 600 RPM. The important point is that most faults are cleared in less than 100 milliseconds. This corresponds to 6 revolutions of a 3600 RPM motor, 3 revolutions of an 1800 RPM motor, and 1 revolution of a 1200 RPM motor.
The next consideration is motor application. Pump and fan motors slow down when input power is reduced. The intersection of the motor torque curve with the fan or pump curve transitions to a new operating point, as illustrated in Figure 4. Compressors and other high torque motors stall when input power is reduced.

The final consideration is motor reacceleration. As soon as the fault is cleared, there is an immediate, significant increase in load as many motors attempt to simultaneously reaccelerate to their pre-fault speed. The electric power grid can produce 110% bursts of power for short periods of time. If reaccelerating motors require more power than the power grid can provide, as shown in Figure 5, then a wide area blackout is likely to occur.
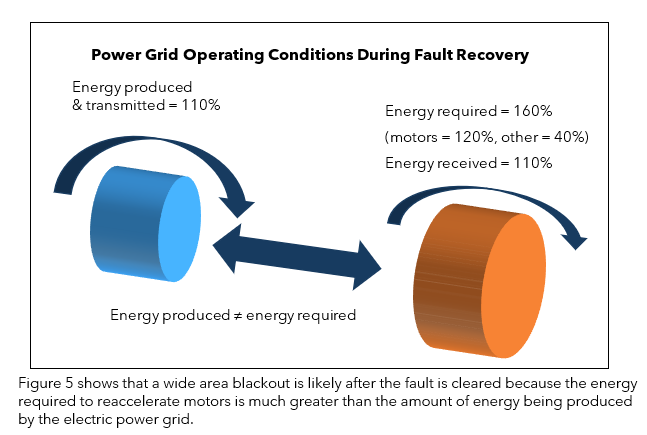
After the Fault
Reacceleration of the electrical grid is referred to as Fault Induced Delayed Voltage Recovery (FIDVR). If grid voltage does not quickly recover, within 10 seconds, auxiliary systems in energy production facilities will become unstable and lead to grid collapse. This phenomenon is illustrated in Figure 6 at twenty seconds after a fault is cleared.
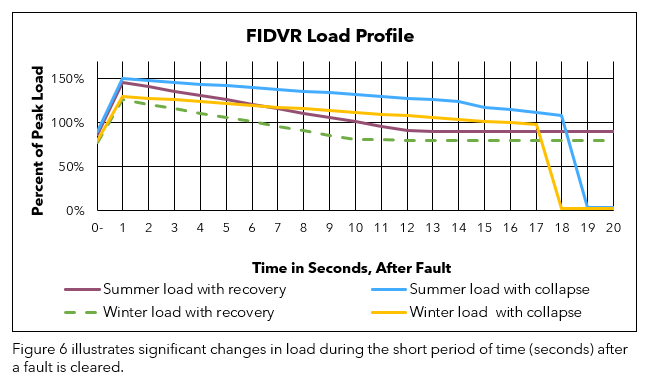
A detailed analysis of this 20-second window is key to preventing wide area blackouts. Energy providers need to provide detailed, seasonal, time sensitive models that include real power output (KW), excitation energy output (KVAR), VAR limits, and machine inertia to transmission system operators. Electric utilities need to provide detailed system diagrams that include seasonal customer load and renewable energy sources at each substation. Seasonal customer loads need to be categorized as: resistive, constant power, general purpose motors, and high torque motors. Renewable energy sources need to be categorized as machine-based sources or power electronics-based sources.
Transmission system operators need to develop seasonal, bifurcated FIDVR models to determine whether grid collapse is likely when a three phase fault occurs. Figure 7 is a representation of grid voltage from NERC’s assessment of the Washington, D.C., Area Low-Voltage Disturbance Event of April 7, 2015. This shows that voltage in Washington, D.C., was 82% of nominal when a fault occurred at a location that was 30 miles away.
Figure 7 shows a recoverable FIDVR event that can be analyzed using traditional load flow analysis methodology. If grid voltage drops to less than 75% of nominal, bifurcated, dynamic system modelling is needed.
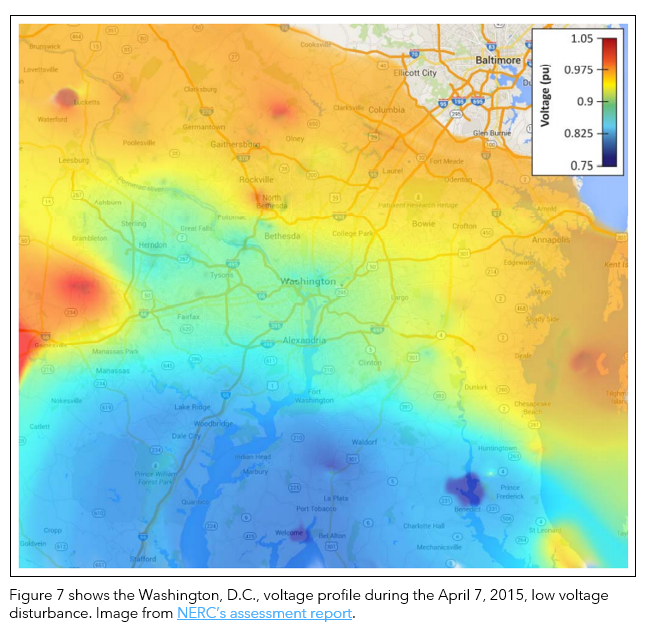
Triggering Event Analysis
To perform an analysis of a triggering event that may lead to a wide area blackout, the following steps are key:
1. Develop wide area voltage profiles, similar to Figure 7, for faults at locations in the electric power grid.
2. Assign fault clearing times, 100 milliseconds for normal fault clearing, 250 milliseconds for stuck circuit breaker clearing.
3. Estimate the percentage of fans and pumps that:
Trip offline because of the fault induced voltage dip.
Slow down because of the fault induced voltage dip.
Reaccelerate when voltage recovers.
4. Estimate the percentage of high torque loads that:
Trip offline because of the fault induced voltage dip.
Stall because of the fault induced voltage dip.
Reaccelerate when voltage recovers.
Transmission System Voltage
As transmission voltage increases, risk increases. System voltage as a function of fault location is shown in Figure 8 for a system that has one transmission line between substations. The significance of Figure 8 is: at 765 KV, faults that are within 35 miles of a substation can become triggering events; and at 230 KV, faults need to be within 10 miles of a substation to become a triggering event.

Figure 8 illustrates data presented in short circuit calculations. This data needs to be converted to voltage profiles as shown in Figure 7 when analyzing the risk of a wide area blackout.
Figure 9 shows substation voltage as a function of fault location for a system that has two transmission lines between substations.

The significance of Figure 9 is: at 765 KV, faults that are within 50 miles of a substation can become triggering events, while at 230 KV, faults need to be within 20 miles of a substation to become a triggering event.
In our next post in this series on wide area blackouts, we’ll be talking about energy sources capabilities. Through risk assessment and implementation of mitigation techniques, the risk of wide area blackouts can be significantly reduced. To learn more about Prescient’s wide area blackout risk assessment service, or to discuss the concept of wide area blackouts in greater detail, contact us.
Comments